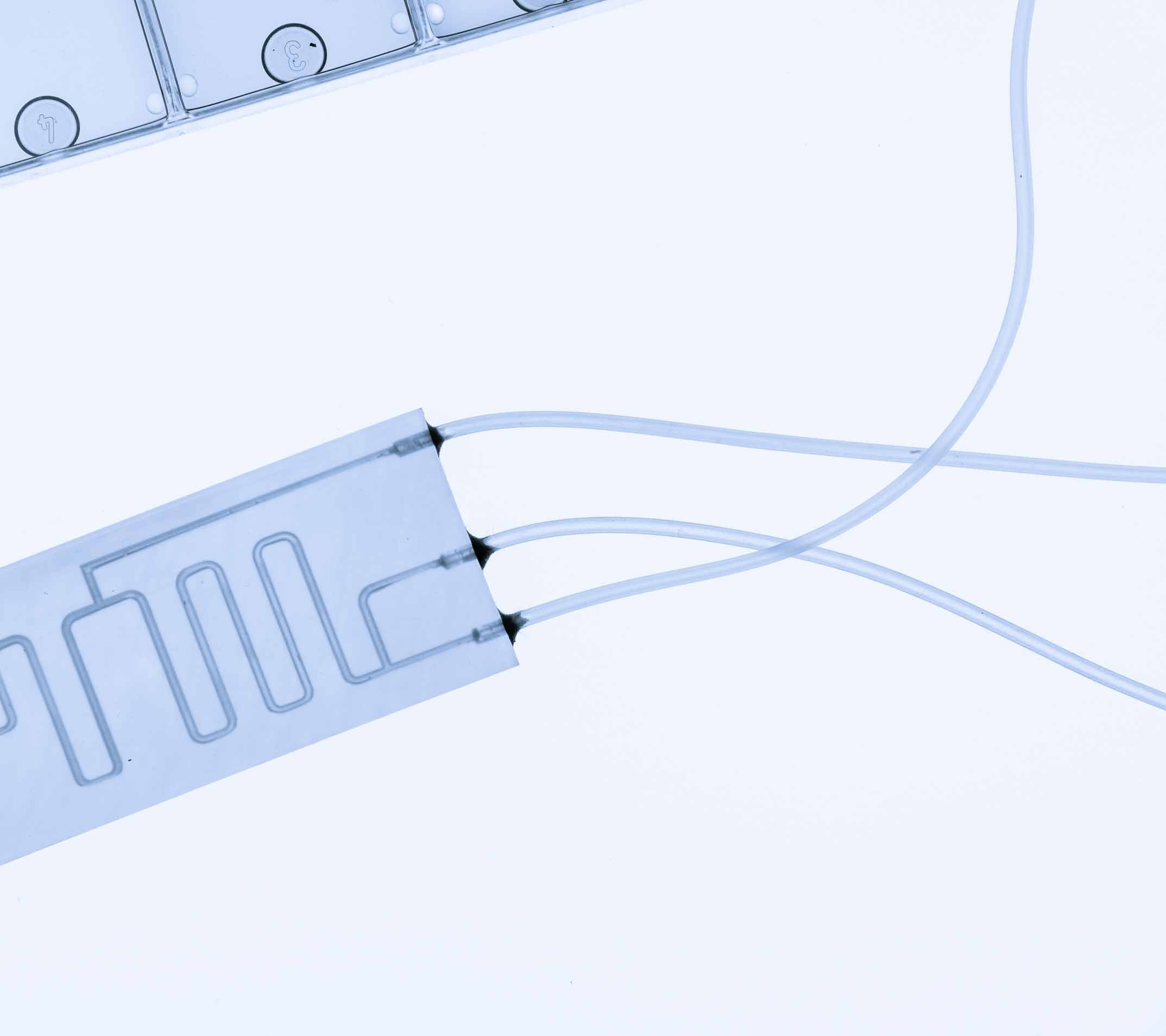
The development of drugs can be a slow process due to the need for safety and extensive testing. Before a drug can proceed to human trials, it is important to ascertain information relating to efficacy and toxicology. Specialised mathematical methods such as pharmacokinetic modelling may be used to predict the ongoing effects of drug dosing over time, and to track changes in drug concentration. However, these models are still reliant on external data such as detailed anatomical and physiological information. Historically, this data is collected through animal testing. Not only are ethical considerations making animal testing increasingly controversial, but there are also disparities between animal and human studies which can impact the safety and results of the trails. For these reasons, there have been efforts to produce engineered tissue that responds in a similar way to human tissue for trialling new drugs.
Initially, two-dimensional (2D) systems were developed for this purpose. While conventional 2D systems are straightforward to produce, the resulting tissue does not represent the target human tissue particularly well due to the substantial limitations of rigid 2D substrates. Another type of tissue-analog is “organoids”, where cells are left to self-organise into different cell types. While the resulting 3-dimensional structure does better to represent the target tissue, there is very little control over the resulting cell architecture. Therefore, organoids are still not ideal for accurate representation of complicated human tissues.
Organ on a Chip (OoaC) is an exciting new approach, which comes in the form of micro-devices that imitate human organs, including their function and reactions to external stimuli[i]. OoaC is characterised by certain key features. Firstly, the presence of a three-dimensional (3D) arrangement of the tissue on platforms. Secondly, the presence of multiple types of cell in order to better match the tissue being imitated. Thirdly, the modelling of biomechanical forces within the tissues. Through the combination of these aspects, the in-vivo environment may be better simulated, including the structure and interactions between different types of tissue. As a result, it is possible to perform studies more accurately on the toxicity of drugs, and the modelling of disease[ii].
OoaC is a young field of research, with most of the development occurring within the last decade. An eventual goal of this technology is to combine multiple artificial organs into one system (known as “body-on-a-chip”) to capture the complex interactions between organs.
The Technological Landscape
Several human organs have been modelled with OoaC, such as brain, skin, kidney, bone and cartilage. In addition to those, key features of promising OoaC technology are summarised below.
Lung-on-a-chip
A significant piece of early OoaC research successfully replicated in-vivo responses of the alveolar-capillary interface, including the biomechanical stretching forces[iii]. This was achieved by growing alveolar epithelial cells and pulmonary microvascular endothelial cells on opposite sides of a porous membrane. To validate the accuracy of the tissue, it was observed that inflammation of the cells occurred under a particular stimulus, and that the system reacted in response to infection with E-coli bacteria. Other studies into lung-on-a-chip have examined the effects of bacteria and cigarette smoke[iv].
Heart-on-a-chip
One key feature modelled in cardiac tissue is the response to electrical impulses. Using cardiomyocytes in a 3D microfluidic device, it was observed that the device was able to replicate heart arrhythmia and dose-dependent changes in heart rate corresponding to those in human trials[v]. Other parts of the heart such as the valves have also been bioengineered to observe the response to particular hormones and drugs[vi].
Liver-on-a-chip
Recreation of liver tissue is especially important for detoxification studies. Additionally, due to the large number of functions performed by the liver, there are a number of liver diseases that would be more effectively researched using a realistic liver-on-a-chip. To accurately simulate a liver, microfluidic devices are used to imitate the hepatic lobules of the liver[vii]. To date, liver-on-a-chip tissues have been used to assess the production of urea and proteins, and to analyse the effects of alcohol on the tissue. A recent study has also compared liver-on-a-chip for rats, dogs, and humans in order to identify the importance of using human cells in particular trials, and to indicate which trials may still be accurate using non-human tissue[viii].
Gut-on-a-chip
In order to model the gut, an Extracellular Matrix-coated membrane separates two microchannels fabricated from PDMS polymer. The system is also configured to experience peristaltic fluid flow similar to that used in real gut tissue[ix]. Engineered gut tissue may be used to examine how well orally administered drugs are absorbed by the body. Additionally, certain gut diseases such as IBD can be modelled using gut-on-a-chip technology and the effects of particular drugs on its treatment may be observed[x].
The Patent Landscape
Patent applications must contain new and inventive subject matter to be granted. The patent landscape can therefore be a key indicator of a growing field of research and innovation.
In the past 13 years, since the first OoaC patent application was published, there has been a strong upward trend in worldwide patent publications* for OoaC technology, as shown below. From 2009 to 2019 there was strong year-on-year growth in OoaC patent publications, which dropped off slightly in 2019 and 2020. Growth has returned in 2021, however, which demonstrates continued interest and innovation in the OoaC field.
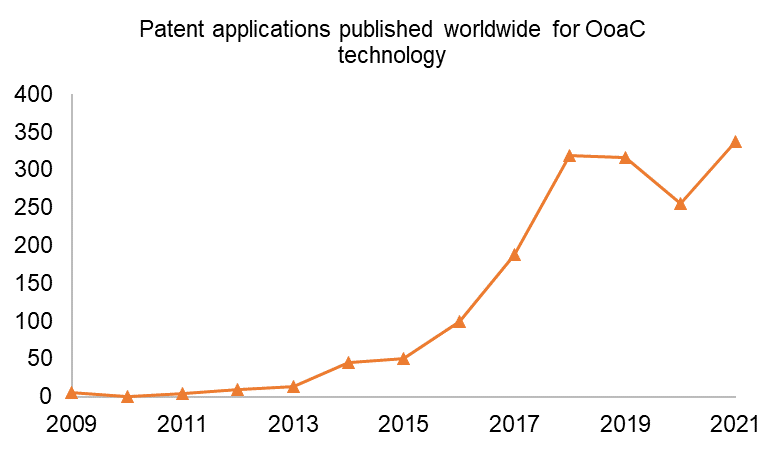
* Patent filing data sourced from Espacenet, July 2022
A relevant comparison point is the number of related scientific papers published worldwide during the same time period. As can be seen below, there has been steady year-on-year growth in the number of scientific papers relating to OoaC technology. This backs up the notion that there is consistent innovation taking place in the OoaC field.
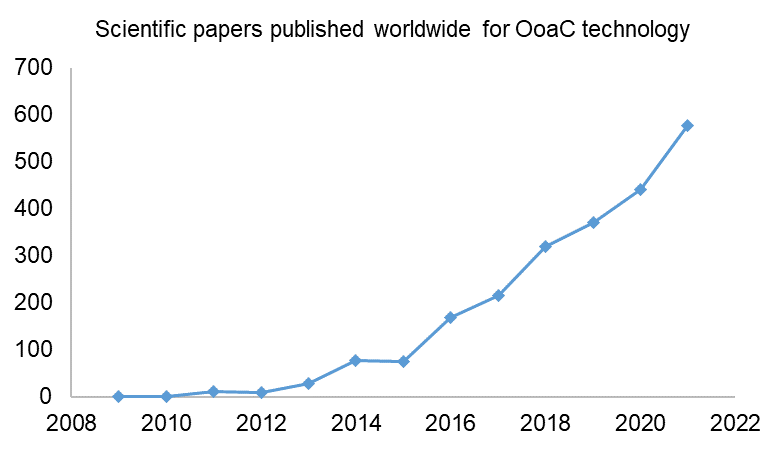
When comparing the number of published patent applications to the number of published scientific papers year-on-year, it is interesting to note that the scientific publications do not have a levelling-off or drop in 2019 and 2020. This could suggest that some potential patent rights might have been missed, with technological advances having been published in journals and not patented. As the subject matter of a patent needs to be new and inventive over what already exists in the public domain, it is important to remember that the publication of even one’s own research in a scientific journal or other source can prevent future patent rights being achieved if the patent application does not pre-date the publication of the scientific paper. This highlights the importance of seeking guidance from a patent attorney as early as possible if you are considering commercialising your innovation.
In terms of patent applicants worldwide, Harvard University has filed the most patent applications directed to OoaC technology, and indeed academic institutions make up a large proportion of the highest filing patent applicants in this sector, with Vanderbilt University and Massachusetts Institute of Technology (MIT) also in the top four.
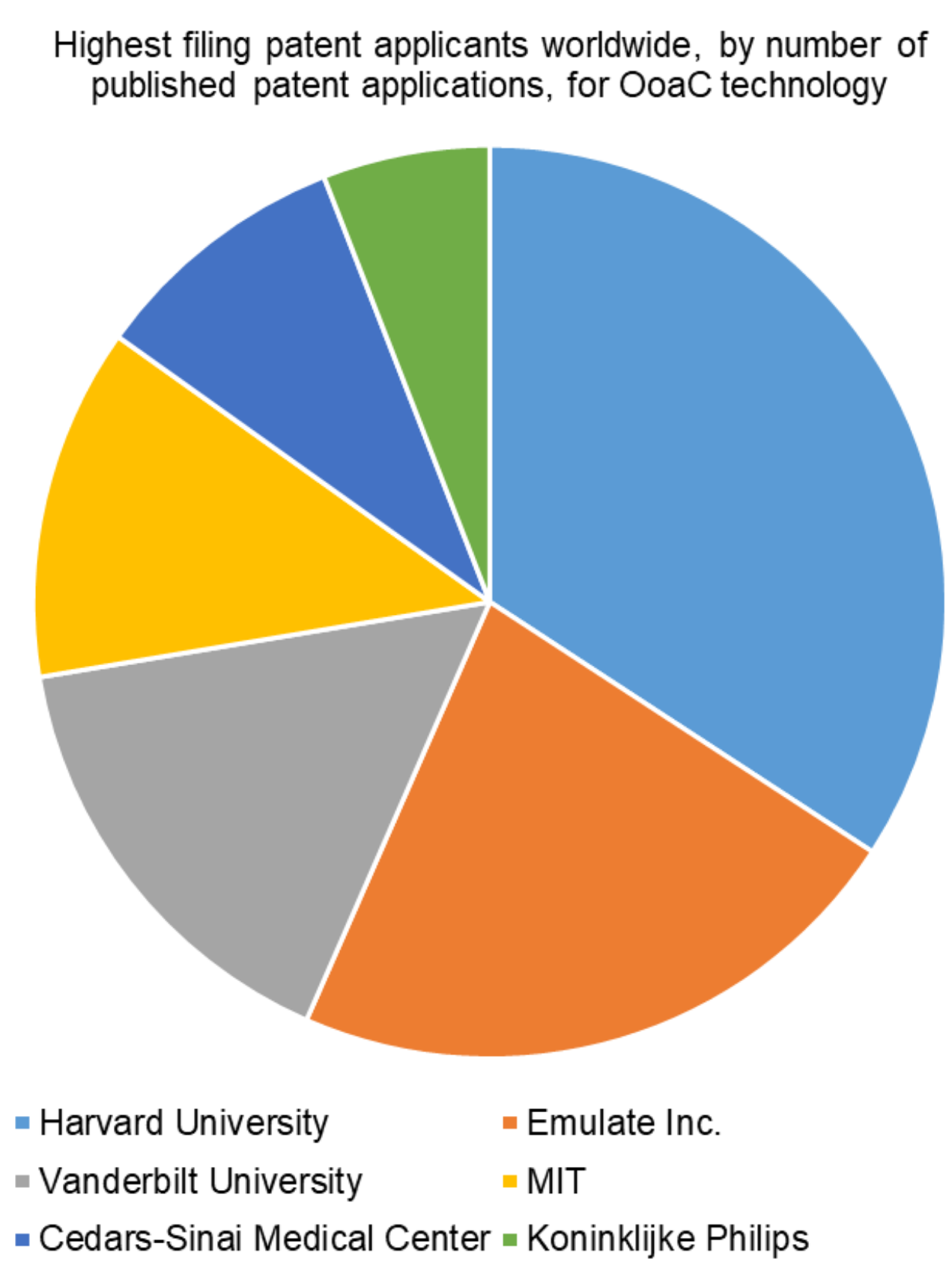
Emulate Inc. is the highest patent filing private company for OoaC technology. It is perhaps therefore not a surprise to learn that Emulate Inc. is a spin-out of Harvard University, based in Boston, USA, which is commercialising OoaC.
Cedars-Sinai Medical Center, in Los Angeles, USA, and the multi-national conglomerate Koninklijke Philips N.I. (also known as Philips) are also notable applicants in the OoaC space.
Turning to the top territories for patent protection, the USA has the highest number of patent applications filed relating to OoaC technology. This might be expected, given that the five highest patent filing applicants are all based in the USA.
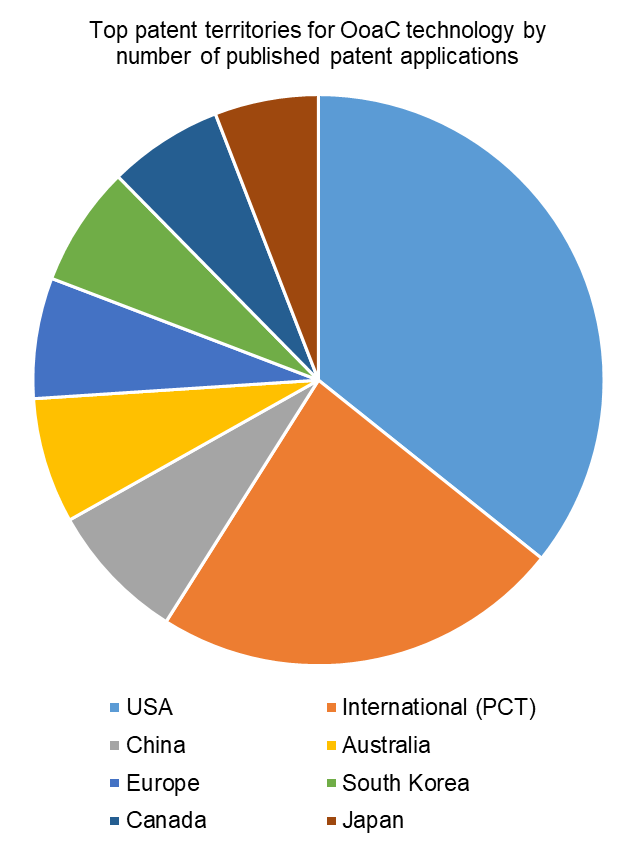
International (PCT) applications make up the second highest filing territory. PCT applications do not provide worldwide patent protection in themselves but are instead effectively ‘holding’ applications, which can be converted to various national and regional patent applications up to two-and-a-half years from their earliest priority date. Those national and regional applications may include all of the other territories shown, so the large proportion of PCT applications being filed is indicative of the commercial interest in securing broad geographical patent protection for the technology, rather than targeting only one or two key markets.
China, Australia, Europe, South Korea, Canada and Japan make up the other major filing territories, with over 100 patent applications relating to OoaC technology in each.
OoaC is clearly a growing field of research and innovation. Companies in the OoaC space, or looking to enter the OoaC space, would be well advised to seek to secure the rights to their intellectual property by engaging a patent specialist to help capture their innovations as patent rights, and thereby grow their patent portfolio to ensure they are maximising their potential to get a return on the investment of their hard work in this rapidly growing space. Likewise, companies operating in this space should investigate whether they are potentially encroaching on the patent rights of others, and thus establish their ‘freedom to operate’ position, before making costly commercial decisions.
If you would like to discuss patenting your innovation, your freedom to operate, or any other IP-related matters, please get in touch with our biomedical engineering team: biomedical.engineering@gje.com. The author’s details can be found here and here.
[i] Huh, D et al. From 3D cell culture to organs-on-chips. Trends Cell Biol 21(12): 745-754. (2011)
[ii] Low, L.A., Mummery, C., Berridge, B.R. et al. Organs-on-chips: into the next decade. Nat Rev Drug Discov 20 345-361 (2021)
[iii] Huh, D., et al. Reconstituting organ-level lung functions on a chip. Science 328, 1662-1668 (2010)
[iv] Benam, K. H., et al. Matched-comparative modelling of normal and diseased human airway responses using a microengineered breathing lung chip. Cell Syst. 3, 456-466.e454 (2016)
[v] Mathur, A., et al. Human iPSC-based cardiac microphysiological system for drug screening applications. Scientific Reports 5, 8883 (2015)
[vi] Capulli, A. K., et al. Acute Pergolide exposure stiffnes engineered valve interstitial cell tissues and reduces contractility in vitro. Cardiovas. Pathol. 25, 316-324 (2016)
[vii] Kane, B. J., et al. Liver-specific functional studies in a microfluidic array of primary mammalian hepatocytes. Analytical Chemistry. 78, 4291-4298 (2006)
[viii] Jang, K.-J., et al. Reproducing human and cross-species drug toxicities using a Liver-Chip. Sci. Transl. Med. 11, eaax5516 (2016)
[ix] Kim, H. J., et al. Human gut-on-a-chip inhabited by microbial flora that experiences intestinal peristalsis-like motions and flow. Lab on a Chip 12, 2165-2174 (2012)
[x] Beaurivage C., et al. Development of a human primary gut-on-a-chip to model inflammatory processes. Scientific Reports 10 (1): 21475